|
Université catholique
de Louvain (UCL-Bruxelles)
Louvain Drug Research
Institute > Cellular and Molecular Pharmacology |
|
Chemotherapy
of the intracellular infection |
Quick
links
|
The
efficacy of the chemotherapy of intracellular infection depends on an
effective cooperation between antibiotics and the host.
Antibiotics must not only penetrate inside the cells and reach the infected
cellular compartments, but also express their activity in the corresponding
environment(s).
Using models of uninfected or infected cells (macrophages and non phagocytic
cells), we study the intracellular pharmacokinetics of antibiotics, their
efficacy against intracellular pathogens localized in different subcellular
compartments, and the modulation of their activity by cytokines.
These research programs are closely linked
to those exploring the cellular toxicity
of antibiotics and the novel
antibiotic targets
|
|
|
|
|
|
Team
- Senior investigators:
F. Van Bambeke, P.M. Tulkens
- Post-doctoral fellows:
W. Siala (2012- ), S. Khandekar (2014- ), E. Drouot (2016-), C. Mark (2016-)
- Doctoral fellows:
A. Anantharajah (2011- ), F. Peyrusson (2014-), K.C. Nguyen (2016-)
- Former investigators:
A. Zenebergh (1983-1990), C. Renard (1984-1988), M.B. Carlier (1988-1990),
B. Scorneaux (1989-1994),Y. Ouadhriri (1993-1999), I. Garcia (1994), I.
Paternotte (1995-2000), H. Chanteux (1999-2003), S. Carryn (1999-2003),
T. Happaerts (2001-2002), C. Seral (2001-2003), M. Barcia-Macay (2003-2007),
S. Van de Velde (2003-2010), A. Olivier (2004-2008), P. Baudoux (2005-2010),
H.A. Nguyen (2006-2009), L. Garcia (2008-2013), S. Lemaire (2008-2013),
J. Buyck (2008-2013), J. Bauer (2010-2011)
Collaborations
- O. Denis, A. Vergison
(Hôpital Erasme et Hôpital
universitaire des enfants "Reine Fabiola"; Université
Libre de Bruxelles)
- B. Devreese and J.
Van Beeumen (Laboratory for Protein
Biochemistry and Biomolecular Engineering; University of Ghent)
- B. Guery (Pseudomonas
aeruginosa Host-Pathogen Translational Research Group; Université
de Lille-2, France)
- Y. Glupzcynski (Laboratoire
de microbiologie des Cliniques
universitaires de l'UCL à Mont Godinne)
- M. Raes (Unité
de recherche en biologie cellulaire et Centre
de spectrométrie de masse; Facultés universitaires Notre
Dame de la Paix, Namur)
- P. Appelbaum, K. Kosowska-Shick
(Hershey Medical Center,
Hershey, PA)
- N. Fridmodt-Moller
(National Center for Antimicrobials & Infection Control, Statens
Serum Institute, Copenhagen, Denmark)
- J. Michiels (Department
of Microbial and Molecular Systems, KULeuven, Leuven, Belgium)
- S. Mobashery (Bioorganic
chemistry and biochemistry; University of Notre Dame, Notre Dame, IL)
- E. Oldfield (Department
of Chemistry and Center for Biophysics and Computational Biology, University
of Illinois, Urbana, IL)
Main current research
programs
General
principles
Intracellular bacterial
infection remains a medical and economical threat in spite of the availability
of a large array of antibiotics potentially active against those organisms
in a-cellular systems. Most failures actually stem from the inability of the
drugs to reach the offending organism(s), and/or to effectively act in the
intracellular environment (see figure). More recently, we also noted the importance
of a cooperation with the natural host defenses and their modulators. Our
aim is to determine the main pharmacodynamic and pharmacokinetic parameters
of antibiotics at the cellular level, and to correlate them with their activities
against intracellular organisms in quantitative models (see Figure 1). We
therefore study, mostly by biochemical and microbiological techniques, the
intracellular fate of antibiotics, and assess their effectiveness against
sensitive bacteria of medical or economical importance. These are selected
on the basis of their capacity to invade and thrive in distinct subcellular
compartments (cytosol, phagosomes,...). We also examine the influence of selected
cytokines on these properties.
These studies have allowed
to describe in detail the cellular properties of many classes of antibiotics
of interest in the context of the intracellular infection (fluoroquinolones,
macrolides, synergistins,beta-lactams, oxazolidinones glyco(lipo)peptides,
ansamycins, ...) in a series of models of uninfected cells and cells infected
with Staphylococcus aureus or epidermidis, Listeria monocytogenes
Legionella spp., Pseudomonas aeruginosa, ...).
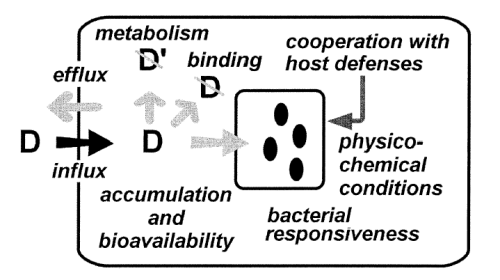
|
Figure
1: Cellular pharmacokinetic and pharmacodynamic parameters governing
the activity of antibiotics in cells
Pharmacokinetic parameters:
- influx
(transmembrane / endocytosis)
- accumulation
and bioavailability (including subcellular localisation, binding
to cellular constituents, metabolic inactivation)
- efflux
(active or passive)
Pharmacodynamic
parameters
- bacterial
responsiveness (fast or slowly growing bacteria, metabolic modulation,
...)
- impact
of physicochemical conditions (pH, other intracellular consttuents,
...)
- cooperation
with host defenses
from
Carryn et al, 2003. |
|
Intracellular
pharmacokinetics
We study the cellular
accumulation (including the mechanisms of entry) and the subcellular localization
of of antibiotics, including of novel molecules in preclinical and clinical
developement, as a basis for further studies examining their intracellular
activities in specific compartments.
The main results of what has been observed over the last 10 years is shown
on Table 1.
In a nutshel, beta-lactams penetrate but do not accumulate in cells, and are
distributed in the cytosol. Macrolides and fluoroquinolones accumulate rapidly
in cells and are distribute primarily in lysosomes and cytosol, respectively.
Aminoglycosides and glycopeptides enter cells by endocytosis and accumulate
specifically in lysosomes. Of note, the level of accumulation of new lipopeptides
like oritavancin can be very high. Linezolid does not accumulate in cells,
but some novel derivatives accumulate to much larger extents.
Selected references on
cellular pharmacokinetics (by reverse chronological order; for a full reference
list, see our publication list)
- Peyrusson F, Butler D,
Tulkens PM, Van Bambeke F. Cellular pharmacokinetics and intracellular activity
of the novel peptide deformylase inhibitor GSK1322322 against Staphylococcus
aureus laboratory and clinical strains with various resistance phenotypes.
Studies with human THP-1 monocytes and J774 murine macrophages. Antimicrobial
Agents and Chemotherapy (2015) 59:5747-5760. (PDF)
- Marquez B, Pourcelle
V, Vallet CM, Mingeot-Leclercq MP, Tulkens PM, Marchand-Bruynaert J, Van Bambeke
F. 2014. Pharmacological characterization of 7-(4-(piperazin-1-yl)) ciprofloxacin
derivatives: antibacterial activity, cellular accumulation, susceptibility
to efflux transporters, and intracellular activity. Pharmaceutical Research
31:12901301 (PDF)
- Lemaire S, Tulkens PM,
Van Bambeke F. 2010. Cellular pharmacokinetics of the novel biaryloxazolidinone
radezolid in phagocytic cells: studies with macrophages and polymorphonuclear
neutrophils.
Antimicrobial Agents and Chemotherapy 54:2540-2548. (PDF)
- Lemaire S, Van Bambeke
F, Tulkens PM. 2009. Cellular accumulation and pharmacodynamic evaluation
of the Intracellular activity of CEM-101, a novel fluoroketolide, towards
Staphylococcus aureus, Listeria monocytogenes, and Legionella pneumophila
in human THP-1 macrophages. Antimicrobial Agents and Chemotherapy 53:3734-3743
(PDF)
- Lemaire S, Van Bambeke
F, Appelbaum P.C., Tulkens PM. 2009. Cellular pharmacokinetics and intracellular
activity of torezolid (TR-700): studies with human macrophage (THP-1) and
endothelial (HUVEC) cell lines. Journal of Antimicrobial Chemotherapy 64:1035-1043.
(PDF)
- Barcia-Macay M, Mouaden
F, Mingeot-Leclercq MP, Tulkens PM, Van Bambeke F. 2008. Cellular pharmacokinetics
of telavancin, a novel lipoglycopeptide antibiotic, and analysis of lysosomal
changes in cultured eukaryotic cells (J774 mouse macrophages; rat embryonic
fibroblasts). Journal of Antimicrobial Chemotherapy 61:1288-1294. (PDF)
- Lemaire S, Van Bambeke
F, Mingeot-Leclercq M-P, Tulkens PM. 2007. Modulation of the Cellular Accumulation
and Intracellular Activity of Daptomycin towards phagocytized Staphylococcus
aureus by the P-glycoprotein (MDR1) Efflux Transporter in human THP-1 macrophages
and Madin-Darby canine kidney cells. Antimicrobial Agents and Chemotherapy
51:2748-2757. (PDF)
- Van Bambeke F, Carryn
S, Seral C, Chanteux H, Tyteca D, Mingeot-Leclercq M-P, Tulkens PM. 2004.
Cellular pharmacokinetics and pharmacodynamics of the glycopeptide antibiotic
oritavancin (LY333328) in a model of J774 mouse macrophages. Antimicrobial
Agents and Chemotherapy 48:2853-2860. (PDF)
Intracellular
pharmacodynamics
We study the activity
of antibiotics in different models of intracellular infection caused
by bacteria sojourning in different subcellular compartments, as illustrated
in Figure 3A for L. monocytogenes(cytosol) and Figure 3B for
S. aureus (phagolysosomes). We then
try to establish their intracellular pharmacodynamic profile (influence of
concentration and of time on activity).
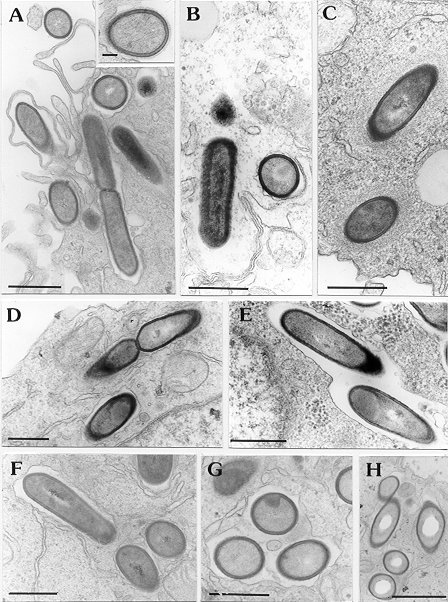
|
Figure 3A:
Following
the intracellular fate of Listeria monocytogenes with electron
microcopy:
Listeria
monocytogenes hly+ (virulent variant) has been phagocytozed
by THP-1 macrophages (A). The bacteria quickly escapes from its
cytoplasmic vacuole (B) to multiply in the cytosol while sourrounding
it-self with a tick layer of finely granular and filamentous material
(actin; C).
In cells pre-treated
with interferon-gamma (D, E), Listeria monocytogenes hly+
remain confined within membrane-bound vacuoles.
Listeria
monocytogenes hly- (non-virulent variant) remains constantly
in vacuoles in control (F, G) as well as in interferon-gamma-treated
cells.
A = 1 h post
infection;
B, C, D, F, G = 3 h post infection
E, H = 5 h post infection
Bars = 1 µ
except for inset of A, where bar is 0.1 µ)
From: Ouadrhiri et al.,1999
|
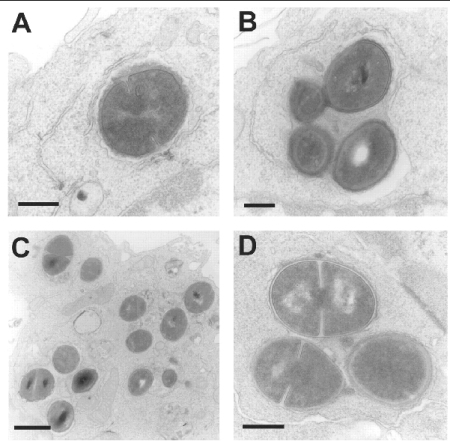
|
Figure 3B: Examining the subcellular localization
of phagocytized S. aureus
Electron microscopy of J774 macrophages fixed 1 h (A and B) or 24
h (C and D) after phagocytosis of opsonized S. aureus. In
both cases, incubation was carried out in the presence of 0.5 mg/L
of gentamicin (to avoid extracellular multiplication of bacteria
released from died cells and subsequent acidification of the medium).
A,B: after 1 h of phagocytosis, bacteria appear isolated (A) or
sometimes in clusters (B) without evidence of damage but with no
sign of division.
C,D: after 24 h of phagocytosis, most of the bacteria are in the
active process of division.
In both cases, all bacteria were seen in membrane-bounded structures
with no evidence of transfer to cytosol. Bars are 0.3 µm (A,
B, and D) and 1 µm (C)
from Seral et al, 2003
|
|
The model allows to determine
3 key pharmacological descriptors of activity, namely
- the maximal efficacy
(Emax; reduction of the intracellular inoculum for an infinitely large concentration)
- the relative potency
(EC50, change in intracellular inoculum half way between no addition of
antibiotic [growth] and Emax
- the static concentration
(Cs; extracellular concentration of antibiotic resulting in no apparent
growth of the post-pagocytosis inoculum
These descriptors allow
to quantitavely assessd the the activity of a given drug against different
intracellular bacteria or different strains with various resistance phenotypes,
and to also to compare difefrent antibiotics.
At the present time, and
using this approach, we are studying antibiotic intracellular activity in
models of phagocytic (monocytes and macrophages) or non-phagocytic (endothelial
cells, keratinocytes, fibroblasts, bronchial epithelial cells) infected by
S. aureus or S. epidermidis, L. monocytogenes, L.
pneumophila, or P. aeruginosa, using both collection strains
and clinical isolates coming from patients with recurrent or persistent infections.
These
studies are leading to largely unanticipated conclusions. Thus, beta-lactams,
which are not accumulated by eucaryotic cells, are actually more active against
the intracellular than the extracellular forms of L.monocytogenes
(Figure 4A).
Quinolones, which do accumulate in cells, show a similar level of maximal
activity (Emax) intracellularly and extracellularly (Figure 4B). Macrolides,
which accumulate to a very large extent in cells, are only bacteriostatic. Some
new glycopeptides, which also accumulate to very large extents but are located
exclusively in the lysosomal compartment, do not act on intracellular L. monocytogenes but are highly bactericidal
against intracellular S. aureus.
The reasons for these
discrepancies are now examined by studying the influence that the intracellular
medium can exert on antibiotic activity (influence of pH or of redox status)
or on bacterial metabolism (using proteomic approaches), to try explaining
the modifications of susceptibility to antibiotics observed intracellularly.
|
Figure
4A
Illustration
of dose-effect relationships in intracellular models of infection.
The left panel shows the influence of time and concentration on
the intracellular activity of ampicillin against Listeria monocytogenes
in infected THP1macrophages exposed for 5 or 24 h to increasing
concentrations of the drug, expressed as the log of multiples of
its minimum inhibitory
concentration (MIC). The right panel provides a comparison of the
dose-effect relationship of the activity of ampicillin and moxifloxacin
against Staphylococcus aureus in infected THP1 macrophages
exposed for over 24 h to increasing multiples of their MIC.
from Van
Bambeke et al, 2006
|
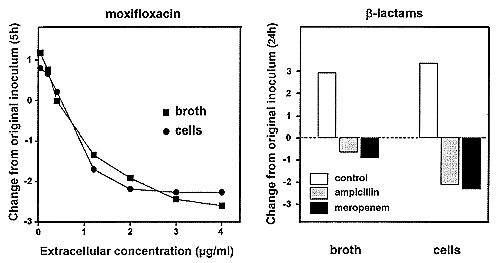
|
Figure
4B
Illustration of some paradoxical
observations made in the model of intracellular infection by
L. moncytogenes. In the left panel, one sees that the
quinolone moxifloxacin shows the same activity against intracellular
and extracellular bacteria despite the fact it is accumulated about
8-fold in the macrophages. In the right panel, one sees that
two beta-lactams, which enter the cells but do not accumulate (cellular
concentration lower than the extracellular one) are essentially
bacteriostatic against L. monocytogenes in broth but become bactericidal
intracellularly after 24 h of exposure of the bacteria to the drugs
from Carryn et al., 2003
|
|
Selected References
on cellular pharmacodynamics (by
reverse chronological order; for a full reference list, see our publication
list)
- Buyck JM, Tulkens PM,
Van Bambeke F. Activity of antibiotic combinations towards resistant strains
of Pseudomonas aeruginosa in a model of infected THP-1 monocytes. Antimicrobial
Agents and Chemotherapy (2015) 59:258-268. (PDF)
- Buyck JM, Tulkens PM,
Van Bambeke F. 2013. Pharmacodynamic evaluation of the intracellular activity
of antibiotics towards Pseudomonas aeruginosa PAO1 in a model of THP-1 human
monocytes
Antimicrobial Agents and Chemotherapy 57:2310-2318. (PDF)
- Melard A, Garcia LG,
Das D, Rozenberg R, Tulkens PM, Van Bambeke F, Lemaire S. 2013. Activity of
ceftaroline against extracellular (broth) and intracellular (THP 1 monocytes)
forms of methicillin-resistant Staphylococcus aureus: comparison with vancomycin,
linezolid and daptomycin. Journal of Antimicrobial Chemotherapy 68: 648658
(PDF)
- Garcia LG, Lemaire S,
Kahl BC, Becker K, Proctor RA, Denis O, Tulkens PM, Van Bambeke F. 2012. Pharmacodynamic
evaluation of the activity of antibiotics against hemin- and menadione-dependent
small-colony variants of Staphylococcus aureus in models of extracellular
(broth) and intracellular (THP-1 monocytes) infections. Antimicrobial Agents
and Chemotherapy 56:3700-3711 (PDF)
- Lemaire S, Kosowska-Shick
K, Appelbaum PC, Verween G, Tulkens PM, Van Bambeke F. 2010. Cellular pharmacodynamics
of the novel biaryloxazolidinone radezolid: studies with infected phagocytic
and nonphagocytic cells, using Staphylococcus aureus, Staphylococcus epidermidis,
Listeria monocytogenes, and Legionella pneumophila. Antimicrobial Agents and
Chemotherapy 54:2549-2559 (PDF)
- Baudoux P, Lemaire S,
Denis O, Tulkens PM, Van Bambeke F, Glupczynski Y. 2010. Activity of quinupristin-dalfopristin
against extracellular and intracellular Staphylococcus aureus with various
resistance phenotypes. Journal of Antimicrobial Chemotherapy 65:1228-1236.
(PDF)
- Lemaire S, Glupczynski
Y, Duval V, Joris B, Tulkens PM, Van Bambeke F. 2009. Activity of ceftobiprole
and other cephalosporins against extracellular and intracellular (THP-1 macrophages,
keratinocytes) forms of Methicillin-Sensitive (MSSA) and Methicillin-Resistant
Staphylococcus aureus (MRSA). Antimicrobial Agents and Chemotherapy 53:2289-2297
(PDF)
- Sandberg A, Jensen KS,
Baudoux P, Van Bambeke F, Tulkens PM, Frimodt-Møller N. 2010. Intra-
and extracellular activity of linezolid against Staphylococcus aureus
in vivo and in vitro
Journal of Antimicrobial Chemotherapy 65:962-973. (PDF)
- Brinch KS, Sandberg A,
Baudoux P, Van Bambeke F, Tulkens PM, Frimodt-Møller N, Høiby
N, Kristensen HH. 2009. Plectasin shows intracellular activity against Staphylococcus
aureus in human THP-1 monocytes and in the mouse peritonitis model. Antimicrobial
Agents and Chemotherapy 53:4801-4808. (PDF)
- Lemaire S, Kosowska-Shick
K, Julian K, Tulkens PM, Van Bambeke F, Appelbaum PC. 2008. Activities of
antistaphylococcal antibiotics towards the extracellular and intraphagocytic
forms of S. aureus isolates from a patient with persistent bacteraemia
and endocarditis. Clinical Microbiology and Infection. 14:766-777. (PDF)
- Van Bambeke F, Barcia-Macay
M, Lemaire S, Tulkens PM. 2006. Cellular pharmacokinetics and pharmacodynamics
of antibiotics: current views and perspectives
Current Opinion in Drug Discovery & Development (2006) 9:218-230.
(PDF)
- Barcia-Macay M, Lemaire
S, Mingeot-Leclercq MP, Tulkens PM, Van Bambeke F.2006. Evaluation of the
Extracellular and Intracellular Activities (human THP-1 macrophages) of Telavancin
vs. Vancomycin against Methicillin-susceptible, Methicillin-resistant, Vancomycin-intermediate
and Vancomycin-resistant Staphylococcus aureus. Journal of Antimicrobial
Chemotherapy (2006) 58:11771184. (PDF)
- Barcia-Macay M, Seral
C, Mingeot-Leclercq MP, Tulkens PM, Van Bambeke F. 2006. Pharmacodynamic evaluation
of the intracellular activity of antibiotics against Staphylococcus aureus
in a model of THP-1 macrophages. Antimicrobial Agents and Chemotherapy 50:841-851.
(PDF)
- Carryn S, Chanteux H, Seral C, Mingeot-Leclercq M-P,
Van Bambeke F, Tulkens PM. 2003. Intracellular pharmacodynamics of antibiotics.
Infectious Disease Clinics of North America (2003) 17:615-634 (PDF)
(UCL only - Copyright owned by the publisher)
- Carryn S, Van Bambeke
F, Mingeot-Leclercq MP, Tulkens PM. 2003. Activity of beta-lactams (ampicillin,
meropenem), gentamicin, azithromycin and moxifloxacin against intracellular
Listeria monocytogenes in a 24h THP-1 human macrophage model. Journal of Antimicrobial
Chemotherapy 51:1051-1052. (PDF)
(UCL only - Copyright owned by the publisher)
- Seral
C, Van Bambeke F, Tulkens PM. 2003. Quantitative analysis of gentamicin, azithromycin,
telithromycin, ciprofloxacin, moxifloxacin and oritavancin (LY333328) activities
against intracellular Staphylococcus aureus in mouse J774 macrophages. Antimicrobial
Agents and Chemotherapy 47:2283-2292. (PDF)
(UCL only - Copyright owned by the publisher)
- Carryn S, Van Bambeke
F, Mingeot-Leclercq MP, Tulkens PM. 2002. Comparative intracellular (THP-1
Macrophage) and extracellular activities of beta-lactams, azithromycin, gentamicin,
and fluoroquinolones against Listeria monocytogenes at clinically relevant
concentrations. Antimicrobial Agents and Chemotherapy 46:2095-2103.
(PDF) (UCL only - Copyright owned by the publisher)
Modulation
of resistance mechanisms in the intracellular environment
In the course of our studies
comparing the intracellular activity of antibiotics against extracellular
and intracellular forms of bacteria harboring typical resistance mechanisms,
we made the unanticipated observation that beta-lactams regain activity against
MRSA when intracellular. This was explained by the fact that the acidic pH
prevailing in the lysosomes where S. aureus sojourns inside the cells
modifies the conformation of PBP2a, allowing for the binding of beta-lactams
(see Figure 5).
We have also shown that
most antibiotics are only poorly and slowly efficient against SCV (Small Colony
variants) of S. aureus, probably in relation with the slow intracellular
multiplication rate of these organisms.
Active efflux is a general mechanism of resistance, with efflux pumps for
antibiotics being expressed at the surface of both eucaryotic cells and bacteria
(see also antibiotic efflux
and permeability resistance mechanisms). We study the cooperation between
efflux pumps expressed by eucaryotic cells and bacteria to reduce the intracellular
activity of antibiotics, as clearly demonstrated for fluoroquinolones and
intracellular Listeria.
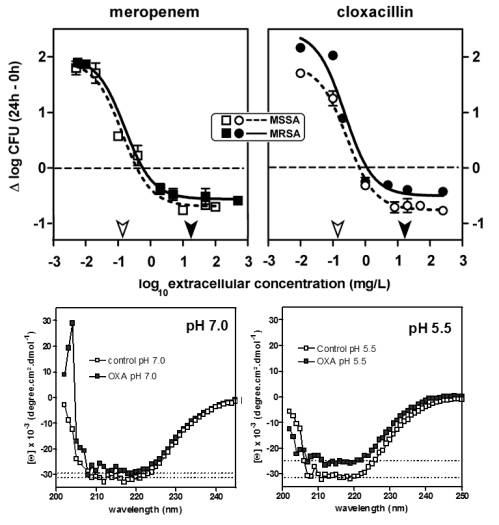 |
Figure
5: Restauration of activity of beta-lactams against intracellular
MRSA, in relation with the change of conformation of PBP2a
at acidic pH
Top:
Concentration killing effects of meropenem and cloxacillin
toward MSSA strain ATCC 25923 and MRSA strain ATCC 33591 after
phagocytosis by THP-1 macrophages. Cells were incubated with
the antibiotics for 24 h at the concentrations (total drug)
indicated on the abscissa. The arrows along the abscissa point
to the MIC of the organisms determined in broth at pH 7.4
(open arrows, MSSA strain ATCC 25923; closed arrows, MRSA
ATCC 33591).
from Lemaire
et al. 2007.
Bottom:
Circular dichroic spectra of PBP2a at pH 7.0 (left) and pH
5.5 (right) in the absence (open symbols) and in the presence
(closed symbols) of oxacillin (30 µM) for 30 min at
25°C. The thin-dotted lines in each graph represent minima
of PBP2a molar ellipticity for each condition.
from Lemaire
et al.,2008.
|
|
Selected References
on expression of resistance mechanisms (by
reverse chronological order; for a full reference list, see our publication
list)
- Garcia LG, Lemaire S,
Kahl B, Becker K, Proctor RA, Tulkens PM, Van Bambeke F. 2012. Intracellular
forms of menadione-dependent Small-Colony Variants of methicillin-resistant
Staphylococcus aureus are hypersusceptible to beta-lactams in a model of THP-1
cells due to cooperation between vacuolar acidic pH and oxidant species. Journal
of Antimicrobial Chemotherapy 67:2873-2881 (PDF)
- Nguyen HA, Denis O, Vergison
A, Theunis A, Tulkens PM, Struelens MJ, Van Bambeke F. 2009. Intracellular
activity of antibiotics in a model of human THP-1 macrophages infected by
a Staphylococcus aureus Small Colony Variant isolated from a cystic
fibrosis patient : 1. Pharmacodynamic evaluation and comparison with isogenic
normal phenotype and revertant strains.
Antimicrobial Agents and Chemotherapy 53:14341442. (PDF)
- Nguyen HA, Denis O, Vergison
A, Tulkens PM, Struelens MJ, Van Bambeke F. 2009. Intracellular activity of
antibiotics in a model of human THP-1 macrophages infected by a Staphylococcus
aureus Small Colony Variant isolated from a cystic fibrosis patient :
2. Study of antibiotic combinations. Antimicrobial Agents and Chemotherapy
53:1443-1449. (PDF)
- Lismond A, Tulkens PM,
Mingeot-Leclercq MP, Courvalin P, Van Bambeke, F. 2008. Cooperation between
prokaryotic (Lde) and eukaryotic (MRP) efflux transporters in J774 macrophages
infected with Listeria monocytogenes. Studies with ciprofloxacin and moxifloxacin.
Antimicrobial Agents and Chemotherapy 52:3040-3046. (PDF)
- Lemaire
S, Fuda C, Van Bambeke F, Tulkens PM, Mobashery S. 2008. Restoration of susceptibility
of methicillin-resistant Staphylococcus aureus (MRSA) to beta-lactam
antibiotics by acidic pH: role of penicillin-binding-protein 2A (PBP 2A) .
Journal of Biological Chemistry. 283:12769-12776. (PDF)
- Lemaire S, Van Bambeke
F, Mingeot-Leclercq MP, Glupczynski Y, Tulkens PM. 2007. Role of Acidic pH
in the Susceptibility of Intraphagocytic Methicillin-Resistant Staphylococcus
aureus Strains to Meropenem and Cloxacillin. Antimicrobial Agents and Chemotherapy
51:1627-1632. (PDF)
Assessment of cytokines-antibiotic cooperation
and antivirulence strategies
Host defenses can modify
the intracellular fate of bacteria and the intracellular activity of antibiotics.
We have studied the influence of gamma-interferon and other cytokines on antibiotic
action against intracellular Listeria monocytogenes, a typical exemple
of food-borne intracellular infection.
In the absence of a sufficiently
powerful cellular immune response, Listeria quiclky speads through
its host. These virulent bacteria gains access to cells by endoyctosis
but escape destruction by egressing from the phagocytic vacuole to reach and
multiply in the cytosol. Interferon-gamma, one of the key cytokine involved
in the immune response against Listeria infection, prevents this evasion
from the phagosome (see Figure 6).
Interestingly, Listeria constrained in the phagosomes become unable
to multiply.
The protection afforded
by the cytokines appears mediated by overproduction of oxygen- and nitrogen-reactive
species. We showed an overexpression of the inducible NO synthase by Interferon-gamma
and IL-6 causes in Caco-2 and by GM-SCF in THP-1 cells, making a direct link
between the immune response and the effective control of Listeria infection.
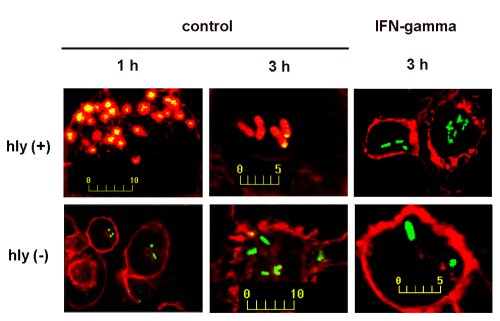 |
Figure 6:
Following the intracellular
fate of Listeria monocytogenes with confocal microcopy.
Listeria,
stained in green with fluorescein-isothiocyanate (FITC) has been
phagocyozed by THP-1 macrophages. The cell actin has been
stained in red with rhodamine-phalloidin. In control cells,
the virulent variant (Listeria monocytogenes hly +), is quickly
surrounded by actin and appears red/yellow, most likely because
bacteria have reached the cytosol. The a-virulant strain (hly -
) remains stained in green. In the presence of interferon-gamma,
bacteria from both strains remain stained in green and presumably
in vacuoles.
From Ouadrhiri
et al. ,1999
|
|
As antibiotic activity
is limited against intracellular infections, we have started to examine other
strategies to control intracellular infection.
A first example is that
of dehydrosqualene synthase
inhibitors
that inhibit the synthesis of staphyloxanthin is S. aureus, a pigment
required for protecting S. aureus against oxydative stress. Strains
producing high amounts of this pigment (like the CA-MRSA US300) show a high
capacity to multiply within the cells, which returns to low values in the
presence of dehydrosqualene
synthase inhibitors
(Figure 7).
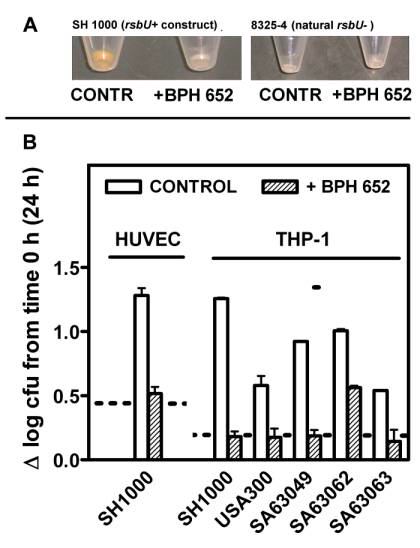 |
Figure 7:
Effects of the dehydrosqualene
synthase inhibitor BPH-652 on staphyloxantin production (top) and
on intracellular growth of different strains of S. aureus (bottom)
A: Bacteria
were grown for 2 days in the absence (CONTR) or in the presence
of the inhibitor (100 µM) before being pelleted for photography
(8325-4 strain subjected to the same treatments is also shown, to
demonstrate the near absence of pigmentation under all conditions).
B: Intracellular
growth of SH1000 in HUVEC cells and of SH1000, USA300, and three
clinical isolates in THP-1 macrophages in the absence (control)
or in the presence of BPH-652. The dotted lines show the response
observed for S. aureus 8325-4 rsbU - (non pigmented strain).
from Olivier
et al., 2009
|
|
Selected
references on cytokine-antibiotic cooperations (by
reverse chronological order; for a full reference list, see the publication
list)
- Garcia LG, Lemaire S,
Kahl B, Becker L, Proctor R, Tulkens PM, Van Bambeke F. 2012. Influence of
the protein kinase C activator, phorbol myristate acetate (PMA), on the intracellular
activity of antibiotics against hemin and menadione auxotrophic Small-Colony
Variant (SCV) mutants of Staphylococcus aureus and their wild-type parental
strain in human THP-1 cells. Antimicrobial Agents and Chemotherapy 56:6166-6174
(PDF)
- Olivier AC, Lemaire S, Van Bambeke
F, Tulkens PM, Oldfield E. 2009. Role of rsbU and staphyloxanthin in phagocytosis
and intracellular growth of Staphylococcus aureus in
human macrophages and endothelial cells. Journal of Infectious Diseases (2009)
200:1367-1370. (PDF)
- Van de Velde S, Nguyen
HA, Van Bambeke F, Tulkens PM, Grellet J, Dubois V, Quentin C, Saux, MC. 2008.
Contrasting effects of human THP-1 cells differentiation on levofloxacin and
moxifloxacin intracellular accumulation and activity against Staphylococcus
aureus and Listeria monocytogenes.
Journal of Antimicrobial Chemotherapy 62:518-521. (PDF)
- Carryn
S, Van de Velde S, Van Bambeke F, Mingeot-Leclercq M-P, Tulkens PM.
2004. Impairment of
growth of listeria monocytogenes in THP-1 macrophages by granulocyte macrophage
colony-stimulating factor : release of tumor necrosis factor-a and nitric
oxide. The Journal of Infectious Diseases 189:2101-2109. (PDF)
- Scorneaux B, Ouadhriri Y, Anzalone
G and Tulkens PM.1996. Effect of recombinant human gamma interferon on intracellular
activities of antibiotics against Listeria monocytogenes in the human macrophage
cell line THP-1. Antimicrob. Agents Chemother. 40, 1225-1230. (PDF)
- Ouadhriri
Y, Scorneaux B, Sibille Y and Tulkens PM. 1999. Mechanism of the intracellular
killing and modulation of antibiotic susceptibility of Listeria monocytogenes
in THP-1 macrophages activated by interferon-gamma. Antimicrob. Agents
Chemother. (1999) 43:1242-1251. (PDF)
- Ouadhriri
Y, Sibille Y and Tulkens PM. 1999. Modulation of intracellular growth of Listeria
monocytogenes in human enterocyte Caco-2 cells by interferon-gamma and interleukin-6:
role of nitric oxide and cooperation with antibiotics. J. Infect. Dis. 180:1195-1204.
(PDF)
Expertise
- Determination of the accumulation
and subcellular distribution of currently available and novel antibiotics
(with mechanistic studies)
- Evaluation of the activity of currently
available and novel antibiotics against intracellular infection (S. aureus,
L. pneumophila, L. monocytogenes, P. aeruginosa, S.
pneumoniae) in models of macrophages and other phagocytic cells
- Analysis of activity
of antibiotics against a large panel of clinical (confirmed cases of pneumonia
and other important infections) and laboratory isolates from these bacteria
with distinct, clinically critical resistance phenotypes
Additional
information: <tulkens@facm.ucl.ac.be>
Last significant update: June 2014